Tracing the Connections Between Agriculture & Water Quality
Nitrate Nexus
A common scene across the Midwestern United States. Nutrients applied to crops can end up in waterways, degrading water quality.
The Raccoon River meanders through the vast farmland outside Des Moines—the state capital and largest city in Iowa—before joining the Des Moines River near the city's center.
The Des Moines River continues snaking its way eastward before flowing into the Mississippi River, a major artery in the U.S. that delivers much of the nation’s nutrient runoff to the Gulf of Mexico.
Ranked as the ninth most endangered river in the United States by American Rivers, the Raccoon River serves as an important source of drinking water for more than half a million people in central Iowa.1
The Raccoon's nitrate levels are among the highest in the nation tied to farming, while a recent dry spell has caused the river to drop to extremely low levels.2
This has caused a city that has historically relied on river water to go into crisis mode and identify other drinking water sources, such as shallow aquifers.2
The Raccoon River serves as a cautionary tale for other cities at the nexus between agriculture and water quality. This article explores this connection, the dark history of industrially-produced nitrogen fertilizers, and the challenges of monitoring nitrate in the field.
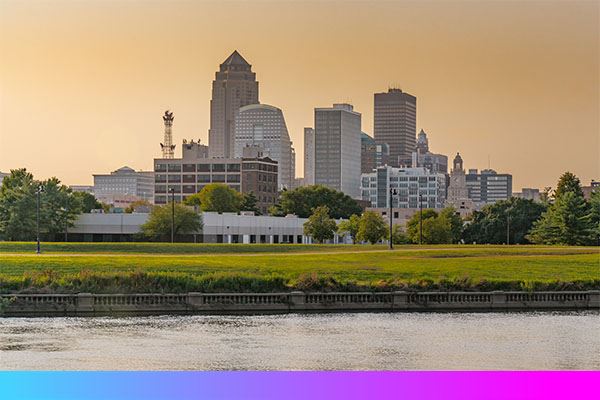
City skyline of Des Moines, Iowa across the Racoon River at sunset.
Feeding The World
Like many from the Midwestern United States, I grew up around flat, seemingly endless fields of corn and soybeans. Farming is one of the most respected professions in this part of the country, and for good reason.
For millennia, farmers have tried to find ways to make land more productive. It's believed our ancestors first recognized the benefits of using manure as a crop fertilizer nearly 8,000 years ago after noticing faster plant growth in areas where animals gathered.3
While the concept of using fertilizer isn't new, it wasn’t until the second half of the 20th century that the widespread use of industrially-produced fertilizers became common.
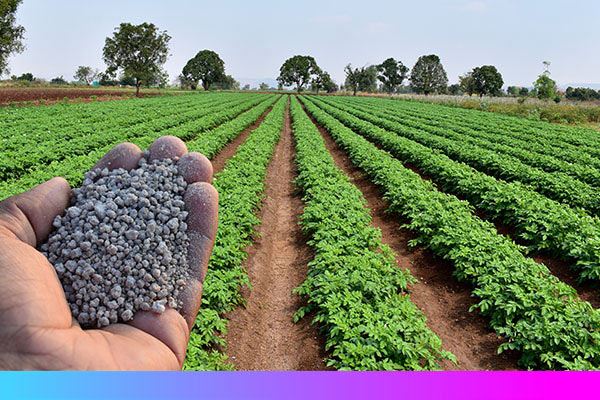
Industrially-produced fertilizers have helped farmers meet the demand of the global population.
Transforming nitrogen in the air into ammonia—the foundation of all synthetic nitrogen fertilizers—is accomplished through the Haber-Bosch process. Fritz Haber developed and demonstrated this process in 1909, and Carl Bosch replicated it on an industrial scale soon after.4
Despite being unfamiliar to nearly anyone that’s not a chemist, this process is one of the single most important chemical reactions ever developed. Without it, our world would be much different.
Between 1900 and 2000, the world’s population quadrupled. Fertilizers were—and still are—a driving factor in this growth, as their use made farmlands significantly more productive. Today, about 50% of the world’s food production relies on fertilizer produced via the Haber-Bosch process.5
The Haber-Bosch process has changed our world—both for the better, and for the worse.
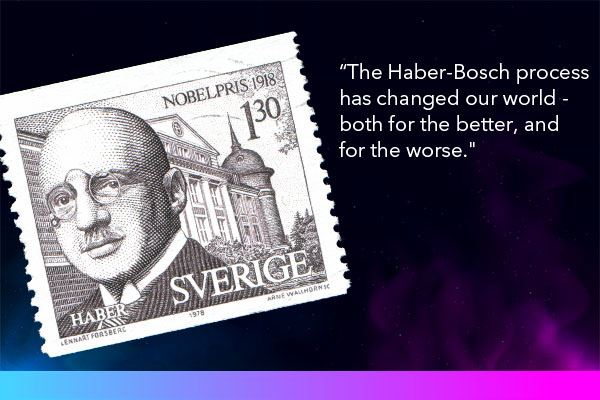
Stamp printed by Sweden, shows Fritz Haber, circa 1978.
So Much For Good Intentions…
Up to 90% of the ammonia produced today from the Haber-Bosch process is used to make fertilizer.5
The rest is used for various purposes, including pharmaceuticals, plastics, textiles, other chemicals—and explosives.5
Mining was once a major source of nitrates, but after the British cut off supplies of nitrates from Chile to Germany during World War I, the Haber-Bosch process kept Germany supplied with fertilizers and munitions.6
Although this was not enough to win the war for the German Empire, it certainly played a role in Germany's ability to continue fighting until the war ended in 1918.
Fritz Haber’s contribution to deadly weapons didn’t end there. While Bosch focused on industry and pure science,7 Haber developed poison gas, even overseeing its first use on the Western Front in 1915.6
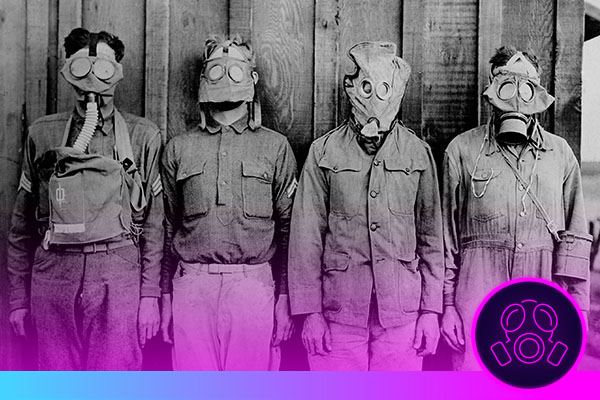
Soldiers wearing WW1 gas masks.
Left-to-Right: American, British. French, German. (1917—18)
In anguish over her husband’s promotion of the new weapon, Haber’s wife ultimately committed suicide. Haber received considerable condemnation from the global community of chemists6 and was given the affectionate name “father of chemical warfare”. 4
Still yet, the Haber-Bosch process is tied to more than war. It’s also connected to our changing climate.
More Than Fertilizer
“In the American Midwest, agricultural runoff is not just inclusive of fertilizer, but also of animal waste,” said Dr. Stephanie A. Smith, our resident microbiology and algae expert at Xylem.
“In states where hog farming is prominent, the waste from confined animal feeding operations (CAFOs) has to go somewhere. It is a significant contributor to nutrients in our waterways. In other parts of the world, it’s human waste.
“In highly arid regions, dust storms can actually deliver nutrients. With the red tides in the Gulf of Mexico, scientists have learned nutrients from dust storms that occur in the Middle East contribute to nutrient enrichment—these ginormous dust clouds traverse the ocean and land in the Gulf of Mexico. It's pretty incredible to think about," added Dr. Smith.
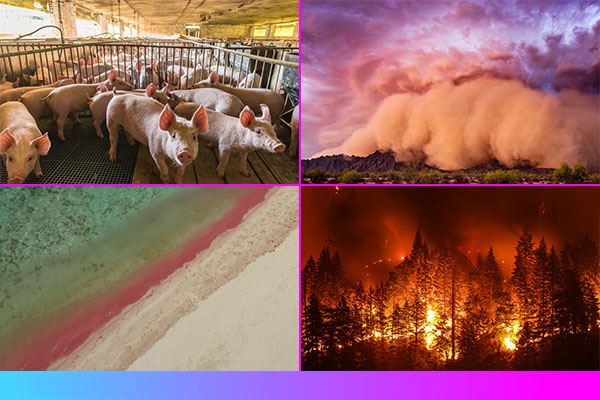
Fertilizer runoff from farm fields isn't the only source of nutrients.
Dust and ash from wildfires are also proving to be contributors to eutrophication. The 2019-2020 Australian wildfires triggered algal blooms in the Southern Ocean downwind of Australia. 8
Other areas of the world—such as the Northwestern United States—have seen similar impacts from intense, devastating fires.
To learn how wildfires negatively impact watersheds, check out our infographic from Mission: Water Issue #7.
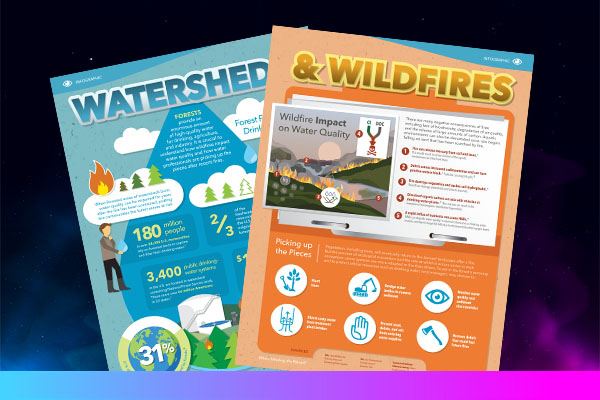
Greenhouse Gas Generation
Greenhouse gases trap heat in the Earth’s atmosphere, driving global climate change. The most prominent of these gases is CO2.
The Haber-Bosch process emits an enormous amount of CO2. In fact, no other industrial chemical-making reaction produces more CO2—it accounts for 1% of global emissions.5
In 2010, 157.3 million metric tons of ammonia were produced by ammonia plants, resulting in the emission of 451 million metric tons of CO2.5 That's a lot of greenhouse gas from a single source!
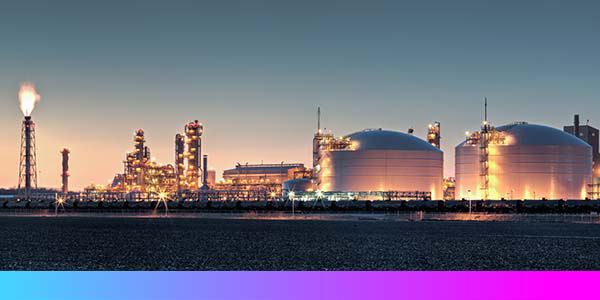
Fertilizer production with the Haber-Bosch process emits tremendous amounts of CO2.
The carbon footprint is so big for two reasons:
1 The process requires a tremendous amount of energy.
2 Hydrogen used for the reaction originates from oil, gas, or coal.
Because of this, some see the ammonia produced via the Haber-Bosch process as a fossil-fuel product. 5
It’s more than just CO2. Nitrate, the breakdown product of nitrogen fertilizers, has wreaked havoc on our water resources.
Water Quality Woes
At the (nitrate) nexus between agriculture and water quality is nutrient enrichment—the main issue I’ve always tied to fertilizer.
Excessive nutrient runoff often results in the eutrophication of surface water. This condition can give rise to harmful algal blooms (HABs) that produce dangerous toxins and cause a drop in dissolved oxygen, resulting in fish kills in both marine and freshwater environments.
HAB events can be dangerous to wildlife and humans, and they can also cause significant commercial and political repercussions.
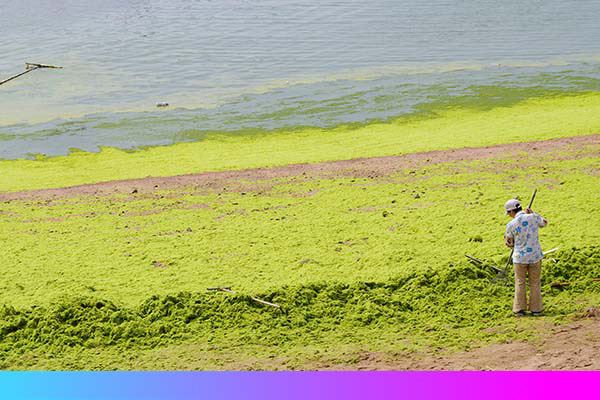
Harmful Algal Blooms (HABs) can be dangerous (or deadly) to wildlife and humans.
Algal blooms in water bodies that serve as drinking water sources are especially problematic. This can result in urgent warnings for citizens to not drink or use their tap water. A commonly cited example of this in the United States is Toledo, Ohio’s water crisis in Summer 2014.
Because of the issues caused by nitrate contamination, I’ve always assumed nitrate monitoring is a key component of nearly every water monitoring program, but this isn’t the case. I spoke with Dr. Stephanie A. Smith, our resident microbiology and algae expert at Xylem, to understand why.
Challenges of Nitrate Monitoring
Dr. Smith clarified that water quality professionals want to measure nitrate directly in our waterways, but it just isn’t that easy.
“Nitrate monitoring in the field has always been a desired thing, but it’s not been easy to do. It’s kind of like coliform testing. It would be awesome if we could just have a real-time view of coliform pollution at our beaches. Well, it’s hard to do,” she said.
Nitrate—and other parameters—have been measured with relative ease in lab settings for decades, but these methods aren’t always field-friendly.
“One of our biggest challenges with water quality monitoring, in general, is instead of building a technology for the field, we’ve always tried to adapt a lab technology to be used in the field.
“I think we’re all starting to appreciate that doesn’t work very well. Unlike in the lab, you can’t control sample preparation in the field,” added Dr. Smith.
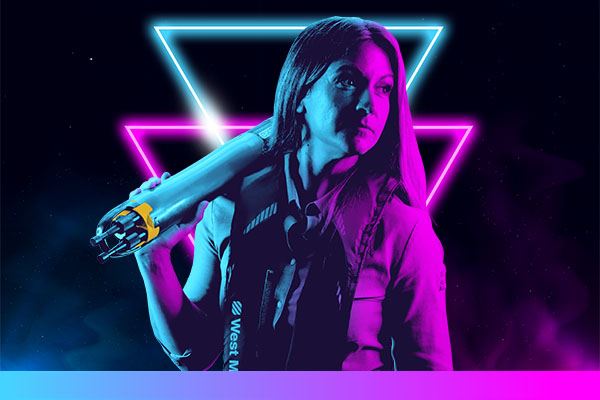
Nitrate has been measured in lab settings for decades, but continuously monitoring nitrate in the field has only recently become feasible.
Issues with Traditional Nitrate Measurement Technologies
Nitrate ion-selective electrodes (ISEs) are available for many water quality platforms, including the YSI ProDSS and EXO. These sensors have a membrane that specifically targets the nitrate ion, but there are issues with using nitrate ISEs in the field, such as interferences.
“Nitrate ISEs are a great example of a lab technology we’ve tried to use in the field. But we’re measuring in the real world, not a controlled setting. There are all kinds of variables to consider,” Dr. Smith pointed out.
Luckily, another type of nitrate sensor is better suited for the field.
Next-Gen Sensing Technology
Nitrate can be detected by measuring the penetration of ultraviolet (UV) light through a solution. This is accomplished using the principles of spectrophotometry.
Those who took physics may remember that forms of electromagnetic radiation (EMR) are distinguished by wavelength. Materials absorb EMR (light) differently, with nitrate absorbing most strongly at short (<250 nm) UV wavelengths.
UV nitrate sensors measure this absorbance of light by nitrate and convert it to a concentration.
UV nitrate sensors are not meant to replace traditional, laboratory-based nitrate analysis methods such as ion-chromatography. Still, they have become an excellent screening tool in the field due to their inherent advantages of being chemical-free and because they don’t require frequent calibration, like ISEs.
Most UV nitrate sensors are large, power-hungry, and expensive, making them impractical for carrying around and for unattended deployment. That all changed with the development of LED-based technology for monitoring nitrate.
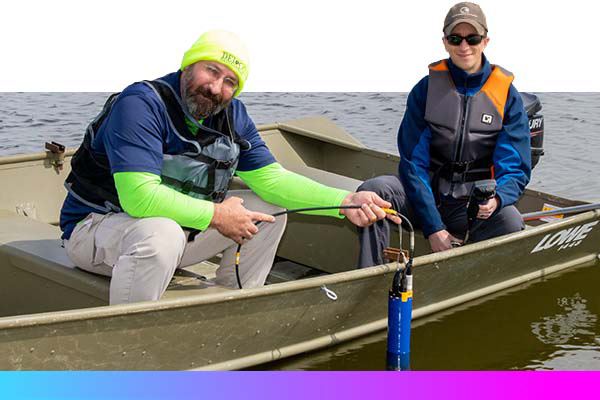
To protect our communities, it's critical to monitor for excessive nutrients in drinking water reservoirs.
Small, But Mighty
Until recently, UV nitrate sensors were large standalone sensors that were not part of a multiparameter platform. YSI’s development of the EXO NitraLED—a small, LED-based sensor that can be installed in the EXO Sonde—was a real gamechanger.
Turns out, being able to simultaneously measure nitrate alongside other parameters is a big deal.
“Measuring nitrate by itself can be interesting—are nitrate concentrations increasing or decreasing? Sure, that's a trend you want to know,” said Dr. Smith.
“But continuous monitoring on a multiparameter platform allows you to do a lot more than just look at the trend of one parameter,” she explained. “It allows you to really evaluate massive data sets.
“So now it’s not about the trend in that parameter—it’s about trends in the system and the relationship between variables. That’s powerful, and that’s the era we’re entering now.”
These environmental systems are complex, so a holistic approach is needed to fully characterize how nitrate impacts aquatic health. This requires the right monitoring tools in the right places.
Nitrate Monitoring, Enlightened
The NitraLED's light source—a small UV-LED rather than a bulky xenon, mercury, or deuterium lamp—
is the secret sauce of the sensor.
“What I think is so revolutionary about NitraLED is that it's not only a deployable technology on a multiparameter platform, it's less expensive,” notes Dr. Smith.
Dr. Smith added that until the NitraLED, the high cost of UV nitrate sensors prevented researchers from deploying a large number of them, hindering their ability to build a more comprehensive understanding of aquatic systems.
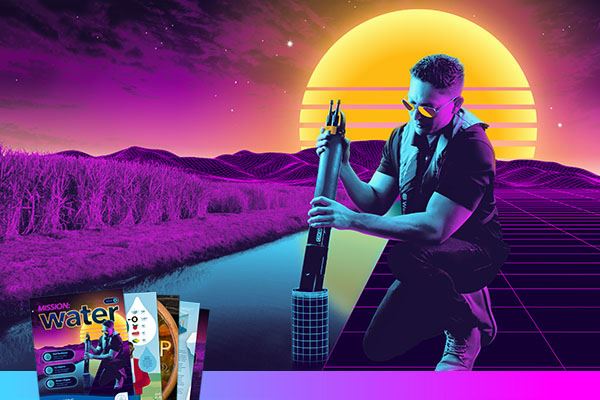
The development of NitraLED is a real gamechanger for nutrient monitoring. The UV nitrate sensor is compact enough to be installed in an EXO sonde alongside other sensors, creating a powerful multiparameter water quality platform.
“Making UV nitrate sensors more affordable is the most recent and exciting step in the evolution of nitrate monitoring,” Dr. Smith said. “What people need is not just to be able to do continuous measurements in situ, they also need continuous measurements at multiple points in a watershed. The lower cost of LED-based nitrate sensors makes this possible.”
Sensors like the NitraLED may be the latest evolution of nitrate monitoring, but NitraLED is not the last step.
Looking To The Future
For many nitrate monitoring programs, the primary goal is to track the contamination source(s) and figure out what to do about it. This requires more than just a new type of nitrate sensor and a massive dataset. Data modeling is the next step, explained Dr. Smith.
“Any program—especially nutrient monitoring programs—should have a modeling component to it. Getting a lot of data is great, but extracting not only backwards but also forward-looking meaning from the data is the future.
“With NitraLED, researchers can continuously monitor for days, weeks, and months, and with these enormous data sets and the power of machine learning, they can not only do source tracking but also do predictive modeling that might prevent future contamination.
“Modeling takes monitoring programs to the next level because it will yield actionable information, rather than just information," added Dr. Smith.
Nitrate sits squarely at the nexus between agriculture and water quality. Monitoring and modeling solutions are needed to better understand this connection—and ultimately find creative solutions to the issue of nutrient enrichment.
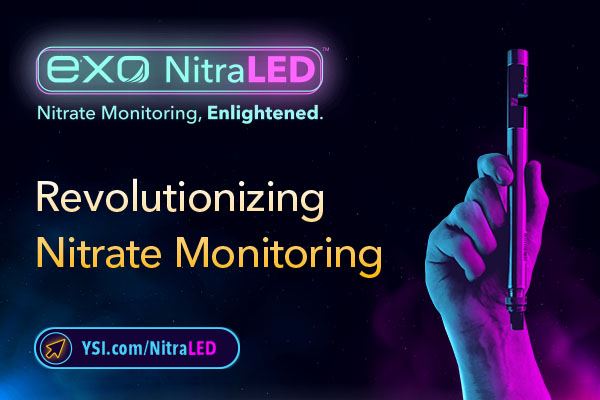
Sources
1. American Rivers,
America’s Most Endangered Rivers - #9: Racoon River, IA
2. Iowa Capital Dispatch,
Water Works pays for Saylorville water too polluted to use, eyes $50M plant expansion
3. University of Nebraska-Lincoln,
A historical Overview of Fertilizer Use
4. BBC,
How fertilizer helped feed the world
5. Chemical & Engineering News.
Industrial ammonia production emits more CO2 than any other chemical-making reaction
6. Science History Institute,
Fritz Haber
7. Nobel Prize,
Carol Bosch Biographical
8. Nature,
Widespread phytoplankton blooms triggered by 2019-2020 Australian wildfires