Safeguarding Water Quality: Managing TOC & THM Risks in Drinking Water Treatment
At the headworks of a drinking water treatment plant, raw water typically undergoes five major processes to become safe for drinking: chemical coagulation, flocculation, sedimentation, filtration, and disinfection. Depending on the characteristics of the source water, additional steps may be necessary to address specific water quality issues, achieve the desired final effluent quality, and manage treatment costs.
Here, we will focus on two critical elements: Carbon and Chlorine. We will explore how the presence of carbon-containing compounds in water influences regulations and treatment decisions and how chlorine, used as a disinfectant, drives additional regulations and treatment decisions.
Figure 1. Drinking Water Process Overview
Carbon
In the Universe, only hydrogen, helium, oxygen, neon, and nitrogen are more abundant than carbon. No one knows when carbon was discovered, but we could attribute that to the first person who handled charcoal from a fire. The element carbon was added to the Periodic Table in 1789. Today, more than one million carbon compounds have been described in chemical literature, and many more are added each day.
Carbon, as organic carbon or Total Organic Carbon (TOC), is an important water quality parameter. In all cases where water is contaminated, many contaminants are carbon-containing compounds. The treatment processes described above are designed to remove these compounds. Some of these compounds naturally exist in the environment. For instance, fulvic and humic acids are formed during the decomposition of plant matter.
Other carbon-containing compounds from industrial or municipal waste can contaminate water. The presence of carbon-containing compounds when chlorine is used as the disinfectant poses a unique set of concerns for operators of water treatment plants due to the potential formation of disinfection by-products, such as Trihalomethanes (THMs).
The first step in water treatment processes is the removal of these compounds. The determination of TOC affords a quick and easy way to measure the amount of organic material that is present in the water and must be removed, and the amount of organic material that has been removed in the treatment process.
Water treatment source water may have TOC levels in the 3 – 5 ppm range and TOC discharge targets of 1 – 2 ppm or less. Municipal wastewater treatment plants may have TOC levels in the 100 – 200 ppm range with discharge targets less than 10 ppm.
TOC and Drinking Water
Strictly speaking, TOC is not regulated. However, there are Congressional Acts and regulatory agencies that require TOC monitoring. These include the Safe Drinking Water Act, the National Pollution Discharge Elimination System, and the Resource Conservation and Recovery Act.
The National Primary Drinking Water Regulations were established under the Safe Drinking Water Act of 1974. Title 40, Parts 141 and 142 I are today's most relevant sections for our discussion. Part 141 establishes the regulations, and Part 142 covers the implementation of those regulations.
Important sections apply to our discussion about carbon – Subpart H systems, the Groundwater Rule, and the Stage 2 Disinfection and Disinfection By-products Rule.
Subpart H systems are public water systems using surface water or groundwater directly influenced by surface water. The Groundwater Rule applies to all systems using groundwater.
The table below shows the required TOC removal for Subpart H systems. The source water TOC range of 2 – 4 mg/L is very typical. As you would expect, as the source water TOC concentration increases, the removal percentage also increases. However, in many cases, a higher percentage of TOC removal beyond the required targets may be beneficial, or necessary, to prevent the formation of disinfection by-products in the distribution system.
Table 1. Required TOC Removal % for Subpart H Systems
The two most important points for the determination of TOC are the Influent and Effluent. However, it is necessary to monitor additional points to adequately map the entire process from the plant to the distribution system. Determination of TOC after sedimentation and filtration is essential. Let's discuss how TOC is determined.
TOC and THM Precursors
A direct correlation between TOC and Trihalomethanes (THM) has been demonstrated in numerous studies. This is particularly true for chloroform and the brominated THMs. There is scientific evidence that THMs can cause short and long-term health effects. If consumed in excess of EPA's standard over many years, some people who drink water containing trihalomethanes in excess of the maximum contaminant level (MCL) could experience liver, kidney, or central nervous system problems and an increased risk of cancer. TOC can remain constant, but reactivity can change. This seems to indicate a change in molecular structure that favors THM formation. This also implies that additional treatment steps may be necessary.
The Building Blocks of TOC Analysis
Every TOC analyzer is comprised of these four building blocks.
Sample Introduction: There must be some mechanism for getting the sample into the analyzer. Some instruments employ direct syringe injection, such as direct sample injection into a combustion furnace.
Oxidation: Simply put, every TOC analyzer oxidizes carbon to carbon dioxide. There are four primary oxidation methods: High-Temperature Catalytic Oxidation, Heated Persulfate, and UV Persulfate.
Detection: Carbon dioxide is measured by one of three detectors: Non-Dispersive Infrared, Conductivity, or Membrane Conductivity.
Display: Lastly, there must be a mechanism for displaying and recording data. In modern instrumentation, it is either an onboard or remote computer.
Figure 2. Basic Building Blocks of TOC Analysis
Heated Persulfate Oxidation with NDIR Detection
The YSI 9220 Total Organic Carbon Analyzer is based on the technique of heated persulfate oxidation with NDIR detection. This technique is compliant with Standard Method 5310C and EPA 514.3. (Watch the YSI 9220 TOC Analyzer/ Overview video).
The persulfate reaction is based on chemical wet oxidation, using sodium or ammonium peroxydisulfate (also simply referred to as "persulfate") and orthophosphoric acid as reactants to convert any carbon-containing organic molecule to dissolved CO2 within a reactor at 98°C.
The 9220 uses sodium persulfate and phosphoric acid. Sodium persulfate is highly soluble in water and forms several radical species that act as strong oxidizing agents.
The chart below shows the redox potential of ions from the highest, fluorine at 2.87 Volts, to the lowest, hydrogen at 0 Volts.
Agent
|
Potential (volts)
|
Fluorine
|
F2
|
2.87
|
Hydroxyl Radical
|
0OH
|
2.80
|
Sulfate radical
|
0SO4-
|
2.60
|
Oxygen radical
|
0O
|
2.42
|
Ozone molecule
|
O3
|
2.07
|
Persulfate
|
S2O82-
|
2.01
|
Hydrogen peroxide
|
H2O2
|
1.78
|
Permanganate
|
MnO4-
|
1.49
|
Chlorine
|
Cl2
|
1.36
|
Dichromate
|
Cr2O72-
|
1.33
|
Chlorine dioxide
|
ClO2
|
1.27
|
Oxygen molecule
|
O2
|
1.23
|
Hypochlorite
|
ClO-
|
0.90
|
Hydrogen
|
H2
|
0.00
|
Table 2. Redox Potential of Ions
In addition to the formation of hydroxyl radicals, other highly reactive radicals are also formed. These include the sulfate radical, SO4-, and the persulfate ion, S2O82-. All these species combine to make persulfate a very powerful oxidizing agent.
Chlorine
Chlorine is a powerful disinfectant used in municipal water treatment processes for over 100 years. From its first use in 1908 in New Jersey to now, it has become the primary disinfectant used in most drinking water and wastewater plants in the United States.1 (Read the blog, When to Measure Free Chlorine vs Total Chlorine | Expert Tips).
Chlorine disinfects water by killing or deactivating many pathogens that lead to disease. Three well-known waterborne diseases that chlorine neutralizes are E. coli, Hepatitis, and Giardia.
Chlorine-based disinfectants provide a more potent residual effect than other disinfectants; therefore, they play a vital role in a municipality's distribution network and remain the disinfectant of choice for drinking water plants. Its use has undoubtedly been a substantial public health benefit in eliminating plagues such as cholera and typhoid and reducing the incidence of intestinal illness and other health problems caused by waterborne germs.
One consequence of using chlorine, and to a lesser degree chloramine, as a disinfectant is that they readily form what are known as disinfection by-products. There are two classes and nine compounds regulated by the EPA: Trihalomethanes and Haloacetic Acids, along with chlorite and bromate. These compounds are formed when chlorine, or chloramine, reacts with the organic carbon and bromide in the water.
In 1998, the Stage 1 DBP Rule was established to reduce drinking water exposure to DBPs. Stage 1 DBP rule established maximum residual disinfectant levels (MRDLs) for three disinfectants: chlorine, chloramines, and chlorine dioxide. Stage 1 DBP Rule also established MCLs for Total Trihalomethanes (TTHMs) at 80 ppb, Haloacetic Acids (HAA5s) at 60 ppb, chlorite at 1,000 ppb, and Bromate at 10 ppb.
In 2006, Stage 2 DBP rule strengthened public health protection by requiring monitoring at sites with the highest DBP levels within the distribution system.
Disinfection By-Products and Regulatory Compliance
The MCLG is the maximum level of a contaminant in drinking water at which no known or anticipated adverse effect on the health of persons would occur, allowing an adequate margin of safety. As noted, MCLGs are non-enforceable public health goals.
The maximum contaminant level (MCL) is the highest level allowed in drinking water based on cost-benefit analysis and is enforceable.
Locational running annual average or "LRAA" means the average of sample analytical results for samples taken at a particular monitoring location during the previous four calendar quarters.
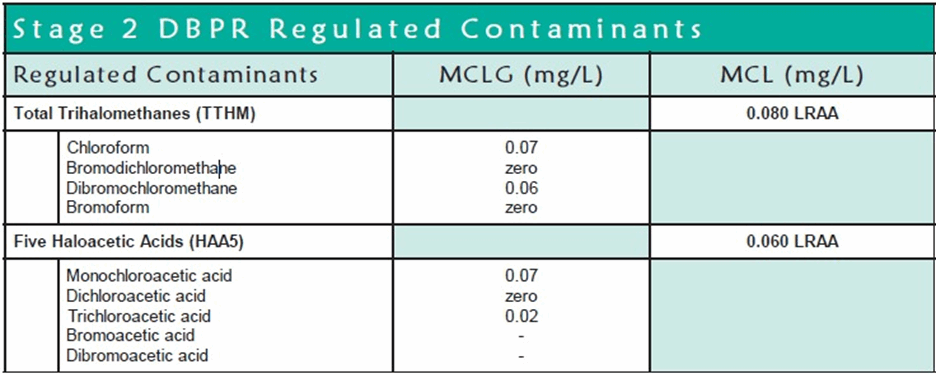
Table 3. Stage 2 DBPR Regulated Contaminants
The total distribution system monitoring points and monitoring frequency are determined by the size of the community and the type of water—either Subpart H or Groundwater.
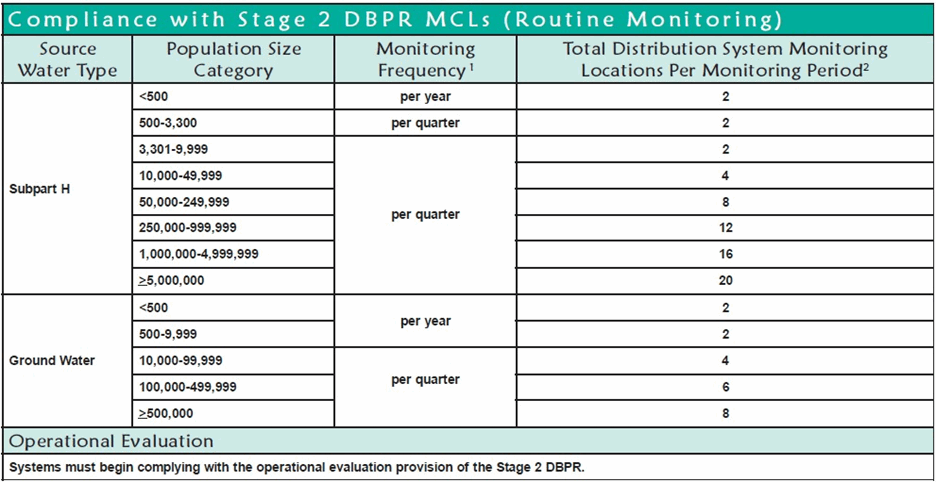
Table 4. Compliance with Stage 2 DBPR MCLs
TTHMs and Drinking Water
The EPA-approved methods for Total Trihalomethanes (TTHMs) are GC/MS or GC/Selective Detector methods. The most commonly used method is 524.2, a laboratory GC/MS method. Compliance with Stage 2 of the Disinfection By-products Rule requires sampling of the distribution system according to the schedule in the above chart.
In addition to the points in the distribution system, it is also helpful to understand the TTHM level in the final effluent leaving the plant before that water reaches the distribution system on a real-time basis. The GC/MS techniques are not readily available for online measurement. However, there is a solution for the real-time determination of TTHMs for the effluent sample.
Online Determination of TTHMs
The YSI 1100 THM Analyzer uses a Purge and Trap/GC-based technique to determine TTHMs. A sample is purged with an inert gas, transforming the purgeable organics to the vapor phase, where it is collected on an absorbent trap at ambient temperature. After the sample is purged, the trap is heated, backflushed, and desorbed with the inert gas onto a chromatographic column. The gas chromatograph is temperature programmed to separate the purgeable organics: chloroform, bromodichloromethane, dibromochloromethane, and bromoform. These compounds are detected by a Surface Acoustic Wave Detector.
Purge State
A sample is purged with an inert gas, transforming the purgeable organics to the vapor phase, where it is collected on an absorbent trap at ambient temperature. After the sample is purged, the trap is then heated, backflushed, and desorbed with the inert gas onto a chromatographic column. The gas chromatograph is temperature programmed to separate the purgeable organics: Chloroform, Bromodichloromethane, Dibromochloromethane, and Bromoform.
Figure 3. Purge Technique to Determine TTHMs
Desorb State
Once the purge step is complete and the analytes have been concentrated on the trap, valves change position, placing the trap in line with the GC carrier gas flow. The trap is rapidly heated to the desorb temperature, releasing the analytes from the adsorbent material, and the GC carrier gas "backflushes" the trap, sweeping the analytes out of the trap through a heated transfer line and over to the GC inlet.
During the desorb and bake states, the sample is drained from the sparge vessel, and the sample lines and sparger are rinsed with clean water (shown in red).
The USEPA methods define this technique, and all commercially manufactured Purge and Trap instruments are based on this principle of operation. Many instruments will include additional elements designed to improve the overall efficiency and performance, but they all have this fundamental backbone.
Figure 4. Desorb State of Purge and Trap Technique
Conclusion
In short, Total Organic Carbon (TOC) plays a significant role in keeping our water safe to drink. The EPA has set clear guidelines for how much TOC should be removed during water treatment, especially for plants that follow Subpart H of 40 CFR. Even when TOC levels are low and steady, organic compounds, or THM precursors, can still be present throughout the treatment process. When chlorine is used as a disinfectant, it can react with these compounds, creating disinfection by-products. That's why closely monitoring both TOC and chlorine levels is crucial to ensure water treatment stays within EPA regulations and keeps our water safe.
References:
- Center for Disease Control and Prevention, Water Disinfection with Chlorine and Chloramine